- Sulfide
- Ether
- Alkoxy group
- Vicinal
- (Vicinal) halohydrin
- Epoxidation
Objectives
- Recognize the structure of ethers and epoxides
- Determine the IUPAC names for simple ethers and epoxides
- Identify several reactions, including synthesis reactions, for ethers and epoxides
Through condensation reactions, we can form ethers from alcohols. The basic condensation reaction is shown below when performed in the presence of sulfuric acid.

We will not discuss sulfides in detail, but you should be aware of this type of molecule. Note that its chemical properties differ from those of ethers.
A chemical compound that is very similar to an ether is an epoxide. Epoxides involve an oxygen and two carbon atoms in a three-atom ring structure, as illustrated below.
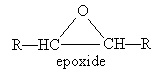
Whereas ethers are relatively stable molecules, epoxides are highly reactive.
Nomenclature
The fundamental functional group for naming ethers is the alkoxy group, several examples of which are shown below with their corresponding names. Note that the names closely resemble the names of the similar alkyl groups.
To properly name an ether according to IUPAC rules, identify the shortest alkyl chain attached to the oxygen atom and consider that portion the alkoxy group. Then follow the usual rules for naming molecules, where the alkoxy group is a substituent of the alkane. Another acceptable naming procedure is to write the two alkyl group names followed by the word ether (similarly to how we named alcohols, such as ethyl alcohol). For example, consider the two molecules below and their acceptable IUPAC names.
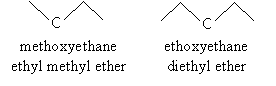
Cyclic ethers have non-systematic names, so we will not focus on this aspect of the nomenclature, but the IUPAC names for several cyclic ethers are given below.
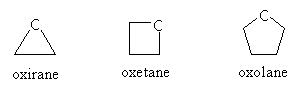
Oxolane also goes by the name tetrahydrofuran.
For epoxides, the functional group name is epoxy when used in the same manner as, for example, ethyl or methyl (that is, following the rules of substitutive nomenclature). When used in the manner of, for instance, ethyl alcohol (functional class nomenclature), the term oxide is used. In the substitutive approach, the epoxy- prefix is preceded by the numbers of the two carbons to which the oxygen is bound. Thus, the following are acceptable IUPAC names for several epoxides.
Also note that because of the structure of epoxies, these molecules can exhibit stereoisomerism. For instance, 3,4-epoxyhexane can actually be either cis-3,4-epoxyhexane or trans-3,4epoxyhexane, as shown below.
In addition to cyclic structures (such as epoxides), ethers can involve multiple oxygen atoms in a carbon chain. For instance, so-called crown ethers are cyclic ethers with multiple oxygen atoms in the ring. An example is shown below, along with its simplified name (we will not discuss nomenclature for crown ethers).
Practice Problem: Determine the IUPAC name for the molecule shown below.
Solution: This compound is 2,3-epoxy-5-methylheptane. Note that the main carbon chain is numbered as shown below.
Synthesizing Ethers and Epoxides
As mentioned previously, we have already studied the acid-catalyzed synthesis of ethers from alcohols. Another method is the Williamson ether synthesis, which involves a reaction between a metal alkoxide and an alkyl halide. For instance, consider sodium ethoxide and bromopropane. The overall reaction is shown below.
The mechanism for this reaction involves dissociation of the metal from the alkoxide and then nucleophilic attack (SN2) on the alkyl halide.
The alkoxide ion acts as a Lewis base with respect to the alkyl halide. Again, note that this reaction is a nucleophilic substitution involving two molecules (SN2).

For epoxides, one approach to synthesis essentially follows this mechanism but involves a single molecule in which a hydroxyl group and a halide attached to adjacent carbon atoms (these functional groups are said to be vicinal, and this particular type of molecule is called a vicinal halohydrin). In the presence of a base (such as hydroxide ions), the halohydrin donates the proton (an acid-base reaction) bound to the oxygen atom. Consider the case below of 3-iodobutanol.
The remaining molecule can then dissociate an iodide ion as follows, creating the epoxide.
The product in this case is 2,3-epoxybutane. The stereoisomer of the product depends on the configuration of the starting molecule.
Another method of synthesizing an epoxy is through epoxidation of an alkene. The reaction involves a peroxy acid, a generic example of which is shown below.
Consider the example of cyclohexene. In the presence of peroxyacetic acid, the following reaction yields 1,2-epoxycyclohexane and acetic acid.
Reactions of Ethers and Epoxides
Let's consider a couple reactions that involve ethers and epoxides: in particular, cleavage of ethers by hydrogen halides and acid-catalyzed ring opening of epoxides (two similar reactions).
The first reaction, cleavage of ethers by hydrogen halides, is exemplified (overall) below for the case of ethoxypropane and hydrogen bromide.

The reaction mechanism in this case proceeds in two steps to yield an alkyl halide and an alcohol.
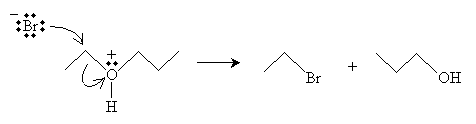
Epoxides can undergo ring opening in the presence of hydrogen bromide in a reaction very similar to that shown above. The mechanism is illustrated below for 1,2-epoxybutane. In the first step, the dissociated bromide ion attacks the less substituted carbon bound to the oxygen atom.
A proton (originating from dissociation of the hydrogen and bromine atoms in hydrogen bromide) then bonds with the oxygen to form 1-bromo-2-butanol.
Practice Problem: Describe the relationship between cleavage of ethers in hydrogen bromide and acid-catalyzed ring opening of epoxides in hydrogen bromide.