- Birch reduction
- Electrophilic (aromatic) substitution
Objectives
- Recognize the effects of benzene's stability on its behavior in reactions
- Learn how benzene derivatives can be synthesized via various mechanisms
- Apply resonance forms to explain the relative stability of benzene-like carbocations
The unique stability of aromatic compounds (arenes) also causes these chemicals to undergo somewhat unexpected reactions, as in the case of bromination of benzene. In this case, electrophilic substitution occurs (in the presence of FeBr3), not the electrophilic addition that we expect for simpler alkenes.

Likewise, benzene (and other arenes) undergoes other interesting reactions, a few representative examples of which we will discuss here.
Birch Reduction
Under high pressure (two to three atmospheres) in the presence of hydrogen and platinum or a similar metal, benzene can be hydrogenated to form cyclohexane. A different reaction, called a Birch reduction, converts benzene into a cyclohexadiene. The reaction takes place in the presence of sodium metal, ammonia (NH3), and a simple alcohol such as ethanol. Note that a single atom of sodium is a free radical because it has one unpaired electron. In addition, sodium readily donates this electron to form a stable eight-electron valence shell. Thus, the sodium radical reacts with benzene as follows.

The anion then acts as a Lewis base in a reaction with ethanol, a Lewis acid in this case.
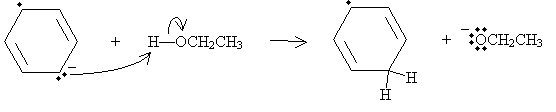
A second sodium atom then donates its unpaired electron, eliminating the radical and leaving another anion structure.
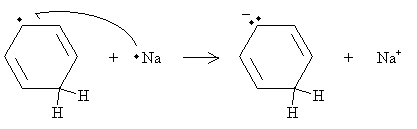
This anion (a Lewis base, as above) reacts with another ethanol molecule (in the same manner as above) to form the final product: 1,4-cyclohexadiene, which is shown below.
Practice Problem: Draw a diagram that illustrates a possible rearrangement of electrons in benzene to form the anion shown in the first step of the Birch reduction above.

In this case, the double bond on the upper right remains unchanged. Using this fact, we can draw the electron movements as shown below, where the single-barb arrows represent movement of one electron and the double-barb arrows represent movement of two electrons.
We saw in our previous discussion of polymers that polystyrene-which you may most easily recognize in the form of Styrofoam (a material used in packaging materials and coffee cups, for instance)-contains a benzene ring. The structure of polystyrene is shown below.
The fundamental unit (monomer) of polystyrene is styrene, again shown below.
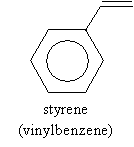
A typical source of styrene is ethylbenzene, which, for industrial processes, is typically produced by dehydrogenation in the presence of zinc oxide (ZnO) at 630°C. This process is shown below.
Practice Problem: Show a reaction mechanism that produces styrene by way of dehydrohalogenation.
Solution: You can choose either the E1 or E2 mechanisms to present a solution to this problem. Here, we simply illustrate the E2 mechanism (that is, an elimination mechanism that involves two molecules). Our starting compound is 1-iodo-1-phenylethane, and the reaction will take place in the presence of sodium methoxide (NaOCH3) and ethanol (CH3CH2OH). Sodium methoxide dissociates into sodium (Na+) and methoxide (-OCH3) ions.
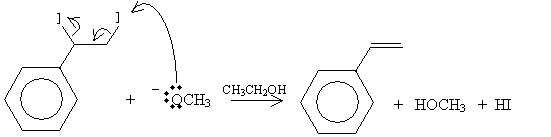
The products in this case are styrene (vinylbenzene), methanol, and hydrogen iodide.
Electrophilic Substitution
Because of benzene's peculiar stability, electrophiles react with arenes in a slightly different manner than we would expect with a typical alkene. The overall addition reaction is repeated below.
If such a reaction were to occur in benzene, the result would be a hexadiene; again, however, benzene's stability rules out this reaction. Assume here that the electrons are distributed in the X-Y compound such that X has a slight positive charge relative to Y. Thus, in the case of benzene, the reaction mechanism begins as follows, where X primarily interacts with a double bond in the benzene ring (that is, it interacts with the ? electrons).
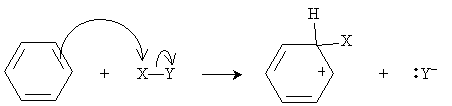
The compound X-Y thus acts here as a Lewis acid, since it accepts an electron pair. (In other words, it is an electrophile.) As we have noted previously, carbocations are highly unstable; nevertheless, because of the resonance forms in this case, some stability is achieved. To increase the stability of the system by reducing its energy, the Y- anion then attacks the hydrogen at the carbon to which X is bound.
Thus, rather than undergoing electrophilic addition, which would have removed the stability of the benzene structure, the arene undergoes electrophilic substitution (or electrophilic aromatic substitution).
An example of electrophilic substitution is chlorination (or, more broadly, halogenation) of benzene. Chlorine is a weak electrophile, so a catalyst is needed. In the presence of iron (Fe), chlorine can form the Lewis acid/Lewis base complex shown below.
This complex acts as an electrophile:
The system is then stabilized when an aromatic ring is formed once more, as the iron (III) chloride anion acts as a Lewis base.
Practice Problem: Show the resonance forms for the carbocation shown in the general electrophilic substitution reaction above.
Solution: The carbocation in this case is more stable because of the resonance forms, which are sketched below. Note that these forms maintain a maximum of four bonds at each carbon site.
Practice Problem: The Friedel-Crafts alkylation of benzene combines benzene and 2-chloro-2-methylpropane and an aluminum chloride catalyst at 0°C. What is a possible route to the product shown below (called tert-butylbenzene)?
Solution: In the same manner as the electrophilic aromatic substitution reaction discussed above, 2-chloro-2-methylpropane can dissociate a chlorine ion, to form a complex with aluminum chloride.
The cation, a strong electrophile, can then attack the benzene ring to form a new combined carbocation.
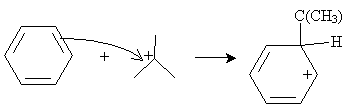
Finally, the anion formed previously can act as a Lewis base to form the desired product.
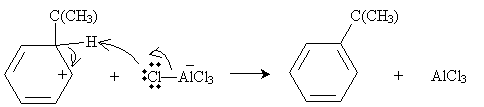